Unpacking the Epigenetics Behind Smoking Mothers and Their Children
- Rithik Kumar
- May 7, 2021
- 6 min read
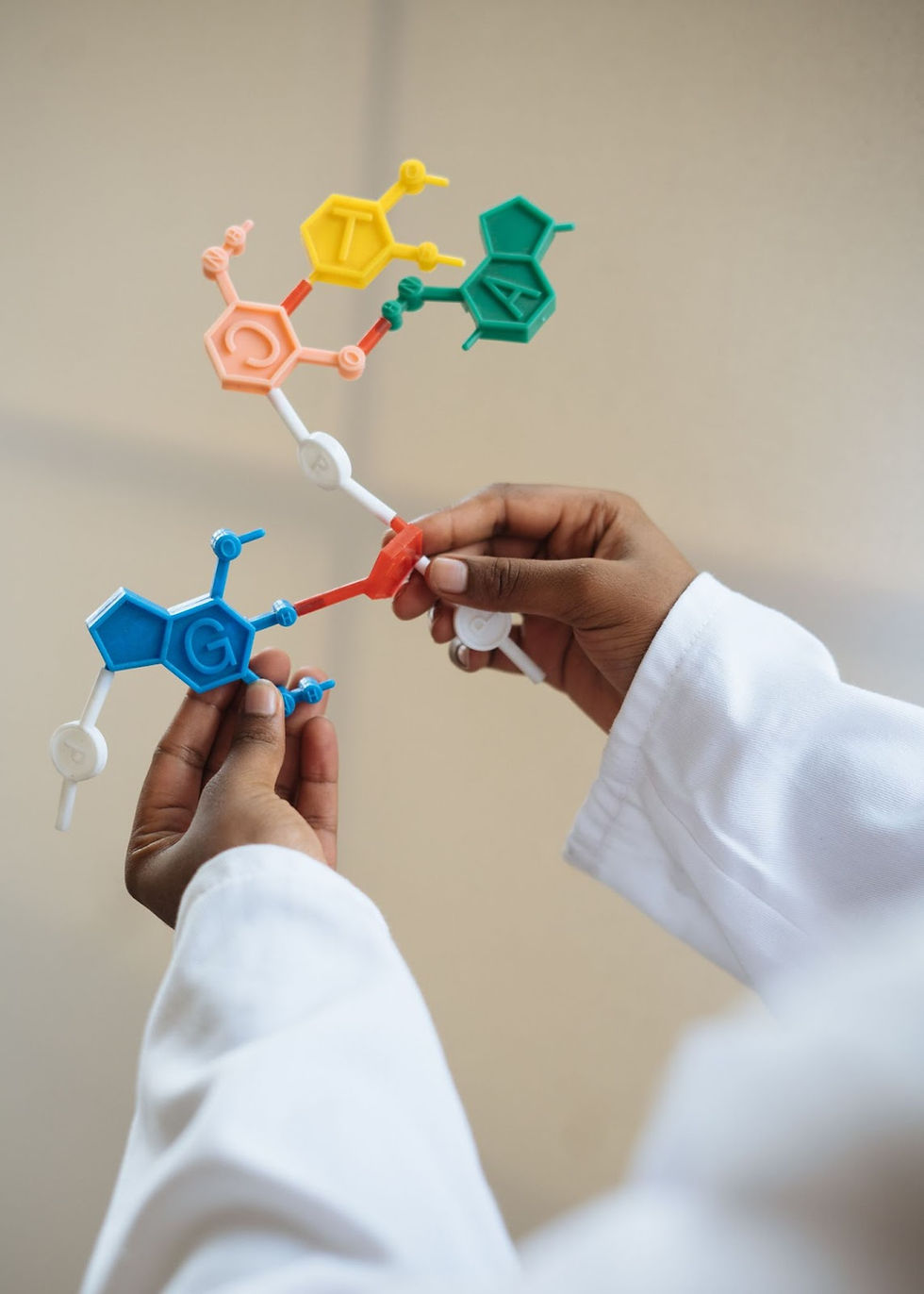
In 2005, the global community adopted an active stance on smoking when governments around the world signed a treaty at the WHO Framework Convention on Tobacco Control. The agreement aimed to severely limit the global demand for tobacco products through a series of targeted economic sanctions (WHO Framework, 2005). Yet, approximately 10.7% of expecting mothers in the United States and up to 40% in Greece continue to smoke during their pregnancy (Tong et al., 2013; Vivilaki et al., 2016). Recent studies suggest that the consequences of maternal smoking may extend beyond an increased disease burden for the mother to that of altered fetal health and development (Joehanes et al., 2016). A retrospective study, conducted by Jaakkola and Gissler, found that even children of light-smoking mothers (10 cigarettes or less per day) presented a 25% higher risk for developing asthma by the age of seven (Schane, Ling, Glantz, 2010; Jakkola, Gissler, 2004). Investigations upon the biological basis of such findings have led to novel research regarding the impact of prenatal tobacco use on the fetal epigenetic landscape. In such studies, researchers utilized 450K epigenome wide array technology to measure association between cotinine levels and DNA methylation found in cord blood samples. Relevant CpG sites were then assessed using statistical tools for significance such as the Bonferri threshold and the false discovery rate to determine genomic regions warranting further analysis. Despite rigorous testing and data analysis, contemporary research has failed to properly assess the entirety of the human genome. By conducting epigenome wide association studies (EWAS) via 450K assays, researchers only focused on differentially methylated regions in pre-selected CpG sites and effectively ignored the influence of other regulatory elements such as enhancers on gene expression. To address this knowledge gap, a 2016 study entitled “Environment-induced epigenetic reprogramming in genomic regulatory elements in smoking mothers and their children” performed longitudinal whole-genome bisulfite sequencing (WGBS) to elucidate the holistic epigenetic impact and functional relevance of prenatal smoking on genomic entities historically excluded in previous EWAS assay based research (Bauer et al., 2016).
With this study, researchers hoped to identify where in the genome environmentally driven epigenetic marks were primarily created. As such, they conducted WGBS of whole blood samples for smoking and non-smoking mothers and their children. After sequencing the samples and adjusting for heterogeneity in blood cell type, 8,409 and 9,743 significant differentially methylated regions (DMRs) were found in children and their mothers respectively, in the comparison of smoking and the non-smoking individuals at the time of birth. About twice as many of these DMRs were located in enhancer as opposed to promoter regions of the genome. And RNA sequencing analysis also revealed a stronger correlation between target gene transcription and differential methylation in regulatory elements, such as enhancers, as opposed to promoters. Accordingly, researchers focused on the JNK2 commuting enhancer, which influences early inflammatory response and the WNT signaling pathway, when attempting to validate differences in DNA methylation among smoking and non-smoking mothers.
Upon investigation, researchers concluded that DNA methylation in the JNK2 commuting enhancer was both genetically and environmentally influenced. Inspection of the genetic landscape near the enhancer revealed a C 🡪 A polymorphism at r55901738. This specific SNP was associated with the creation of a binding site for the Oct4 transcription factor, which when bound to the Oct4 found in children and mothers could decrease DNA methylation in the enhancer (Zimmerman et al, 2013). As a result, children of smoking mothers, who displayed genetically higher levels of the C 🡪 A polymorphism, experienced a negative correlation between genotype and methylation. In addition, differential methylation in the JNK2 enhancer was also a direct product of the fetus’ environmental conditions, specifically maternal smoking habits. Analysis of fetal cord blood revealed an inverse relationship between maternal urine cotinine levels and DNA methylation such that the enhancer was 10% more hypomethylated in children of heavy smoking compared to non-smoking mothers. In order to further validate the causative relationship between prenatal smoking and DNA methylation of the enhancer in the absence of genotypic differences, an in vitro experiment regarding the influence of tobacco smoke extract on peripheral blood mononuclear cells was performed which displayed a similar 11.1% decrease in DNA methylation in 88% of the samples.
Finally, after establishing the combined effect of genes and the environment on differential methylation of the JNK2 enhancer, researchers considered its potential link to adverse phenotype development. As a member of the WNT signaling pathway, the JNK2 commuting enhancer is involved in the conception of various tobacco based lung diseases such as lung cancer and COPD. Differential methylation in the JNK2 enhancer region could therefore have downstream repercussions in regards to respiratory malfunction. Statistical tests revealed significant correlation (P = 0.034, R = -0.46) between DNA methylation of the enhancer and JNK2 transcription in fetuses of smoking mothers. This significant correlation (P = 0.04, R= - 0.16) was also found for the same cohort of mothers and children four years after the initial sampling. Furthermore, logistic regression analysis confirmed, with an odds ratio of 1.40, that DNA methylation in the JNK2 enhancer was associated with an increased risk for wheezing symptoms and thereby also potentially late onset asthma in four-year-old children. Taken together, the multi-year influence of JNK2 enhancer DNA hypomethylation on genetic transcription and the advancement of impaired lung function has striking implications for public health (Bauer et al., 2016).
These findings demand a paradigm shift. By systematically unpacking genetically and environmentally induced DNA hypomethylation in the JNK2 enhancer and its correlation with target gene transcription and adverse phenotype development, researchers insisted that future epigenetics research consider the impact of regulatory elements beyond promoters. Moreover, the consequences of maternal smoking behavior on children can and must be examined via the refined lens of DOHaD (Silveira et al., 2007). By acknowledging the critical role of both the prenatal environment and epigenetic reprogramming on future health outcomes, the global community can work to effectively adopt progressive public health measures and policy. For example, current physician based intervention strategies for smoking in the US involve one-on-one counseling sessions with high-risk groups such as pregnant women. Public health measures should motivate physicians to not only discuss the potentially high likelihood of respiratory distress associated with children of smoking mothers, but also offer opportunities for informed genetic screening for relevant chronic conditions (“Public Health”, 2020). Furthermore, the study indirectly proposes several avenues for future research. Additional experiments are needed to determine the intergenerational persistence of epigenetically driven adverse phenotypes. While the paper primarily focused on the epigenetic deregulation of the JNK2 enhancer, more research is required to understand how other significant DMRs interact with biological pathways similar to WNT. Equipped with an evidence based comprehensive understanding of smoking induced epigenetic regulation, the academic community can finally work to develop targeted demethylation therapies for children during early life.
References
Bauer, T., Trump, S., Ishaque, N., Thürmann, L., Gu, L., Bauer, M., . . . Lehmann, I. (2016). Environment-induced epigenetic reprogramming in genomic regulatory elements in smoking mothers and their children. Molecular Systems Biology, 12(3), 861. doi:10.15252/msb.20156520
Jaakkola, J. J., & Gissler, M. (2004). Maternal Smoking in Pregnancy, Fetal Development, and Childhood Asthma. American Journal of Public Health, 94(1), 136-140.
doi:10.2105/ajph.94.1.136
Joehanes, R., Just, A. C., Marioni, R. E., Pilling, L. C., Reynolds, L. M., Mandaviya, P. R., . . . London, S. J. (2016). Epigenetic Signatures of Cigarette Smoking. Circulation: Cardiovascular Genetics, 9(5), 436-447. doi:10.1161/circgenetics.116.001506
Public Health Focus: Effectiveness of Smoking-Control Strategies -- United States. (n.d.). Retrieved May 28, 2020, from
https://www.cdc.gov/mmwr/preview/mmwrhtml/00017511.htm
Schane, R. E., Ling, P. M., & Glantz, S. A. (2010). The Health Effects Of Light And Intermittent Smoking. D23. Recent Advances On Smoking Health Effects. doi:10.1164/ajrccm conference.2010.181.1_meetingabstracts.a5433
Silveira, P. P., Portella, A. K., Goldani, M. Z., & Barbieri, M. A. (2007). Developmental origins of health and disease (DOHaD). Jornal De Pediatria, 83(6), 494-504. doi:10.2223/jped.1728
Tong, V. T., Dietz, P. M., Morrow, B., D'Angelo, D. V., Farr, S. L., Rockhill, K. M., England, L. J., & Centers for Disease Control and Prevention (CDC) (2013). Trends in smoking before, during, and after pregnancy--Pregnancy Risk Assessment Monitoring System, United States, 40 sites, 2000-2010. Morbidity and mortality weekly report. Surveillance summaries (Washington, D.C. : 2002), 62(6), 1–19.
Vivilaki, V. G., Diamanti, A., Tzeli, M., Patelarou, E., Bick, D., Papadakis, S., . . . Katsaounou, P. (2016). Exposure to active and passive smoking among Greek pregnant women. Tobacco Induced Diseases, 14(1). doi:10.1186/s12971-016-0077-8
WHO Framework Convention on Tobacco Control. (2005). Geneva: World Health Organization.
Kommentare